Overlaps at the Frontiers of Astrophysics, Cosmology and Particle Physics at the XXXIII Canary Islands Winter School of Astrophysics
The type of matter we are made of and we are familiar with accounts only for 5% of the Universe. The rest is the so called Dark Universe, made of something named dark matter (27%), and something named dark energy (68%), two big mysteries.
This year the Winter School of Astrophysics of the Instituto de Astrofísica de Canarias (IAC) has gathered renowned researchers on three fundamental fields: Astrophysics, Cosmology and Particle Physics, which converge into the study of some fundamental questions in particle astrophysics: the nature of dark matter, the nature of dark energy, and related issues such as the weaknesses of the standard model of particle physics. The School tackled these questions with an interdisciplinary approach, nourishing research cooperation across varied ways of obtaining knowledge.
Cosmology studies the observable Universe as a whole using astrophysics’ tools. Thanks to observations, there is data showing that the expansion of the Universe is accelerating. Some astrophysics systems (supernovas, dwarf galaxies, neutron stars…) give information on the possible nature of dark matter and dark energy, which would be linked to the advances in particle physics. From its side, particle physics studies nature at the smallest scales human beings have been able to reach, especially with particle accelerators built on Earth. Smaller scales implies exploring higher energies corresponding to more essential components of the Cosmos. The early universe, an extremely dense plasma, reaches energy levels much higher than the ones generated at a particle accelerator. It is therefore a good “laboratory”.
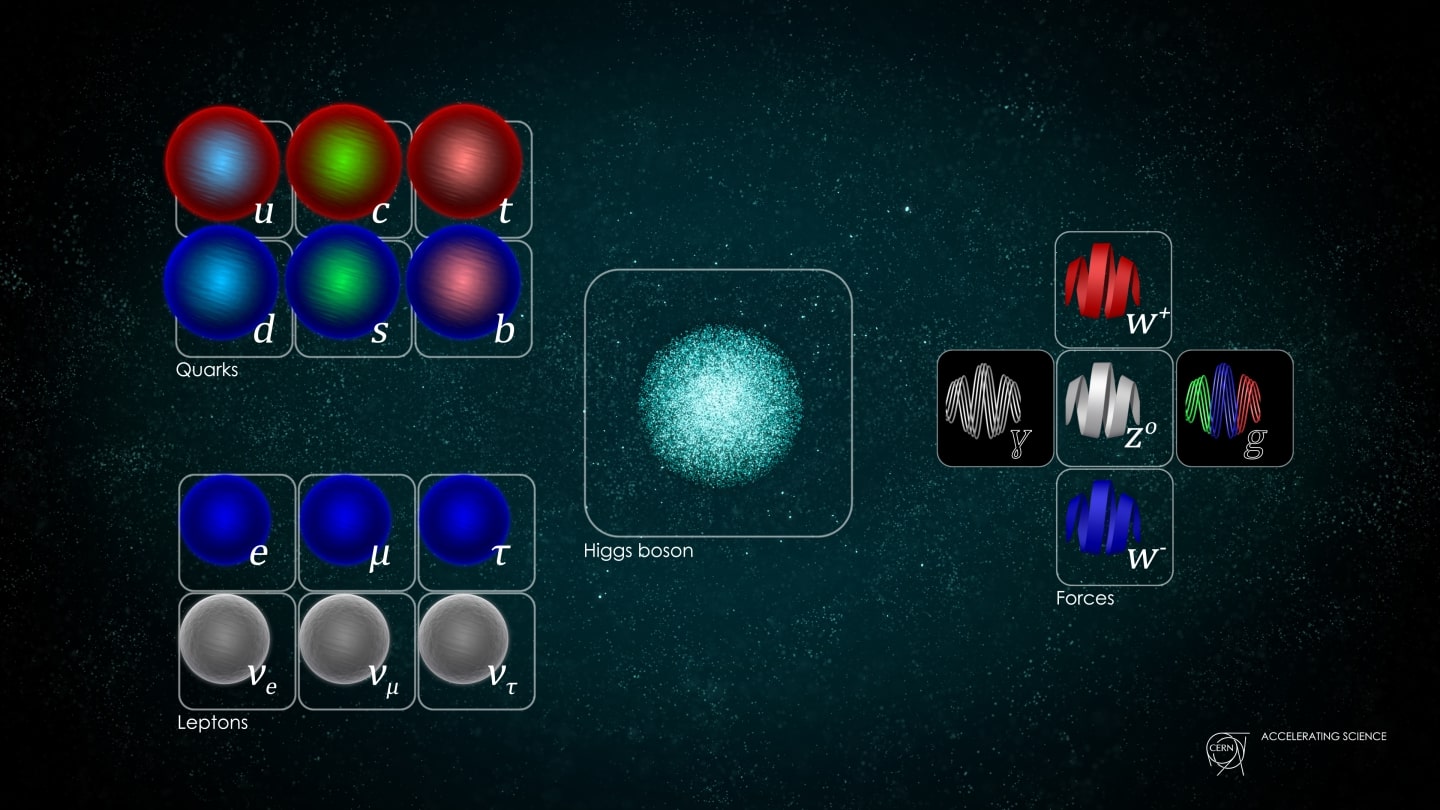
Regardless of each discipline peculiarities, the underlying Physics and Mathematics are always the same. Profesor Kfir Blum (Weizmann Institute of Science) points out that “Our goal is to reduce what we see to a simple expression of reality, this expression should apply to any system. It does not matter if you are looking at the Universe as a whole, as a large system, at a galaxy, or if you are looking at some laboratory experiment. Cosmology, Astrophysics and Particle Physics use some different jargon and terminology, but this is nonsense. If you understand what people are talking about they are always talking about the same things: conservation of energy momentum, general relativity…”
The Universe, a tantalising mystery
The Universe is expanding since its origin, approximately 13.800 millions of years ago. At the beginning, it was an extremely hot plasma. The younger the Universe, the more concentrated and dense, the more energetic. Matter and antimatter are concentrated. If the known laws were applicable from the start, matter and antimatter would have annihilated one another and the Universe would be only radiation. We ignore the physical laws that could be accountable for the primordial asymmetry at the origin of all that humans perceive, including ourselves.
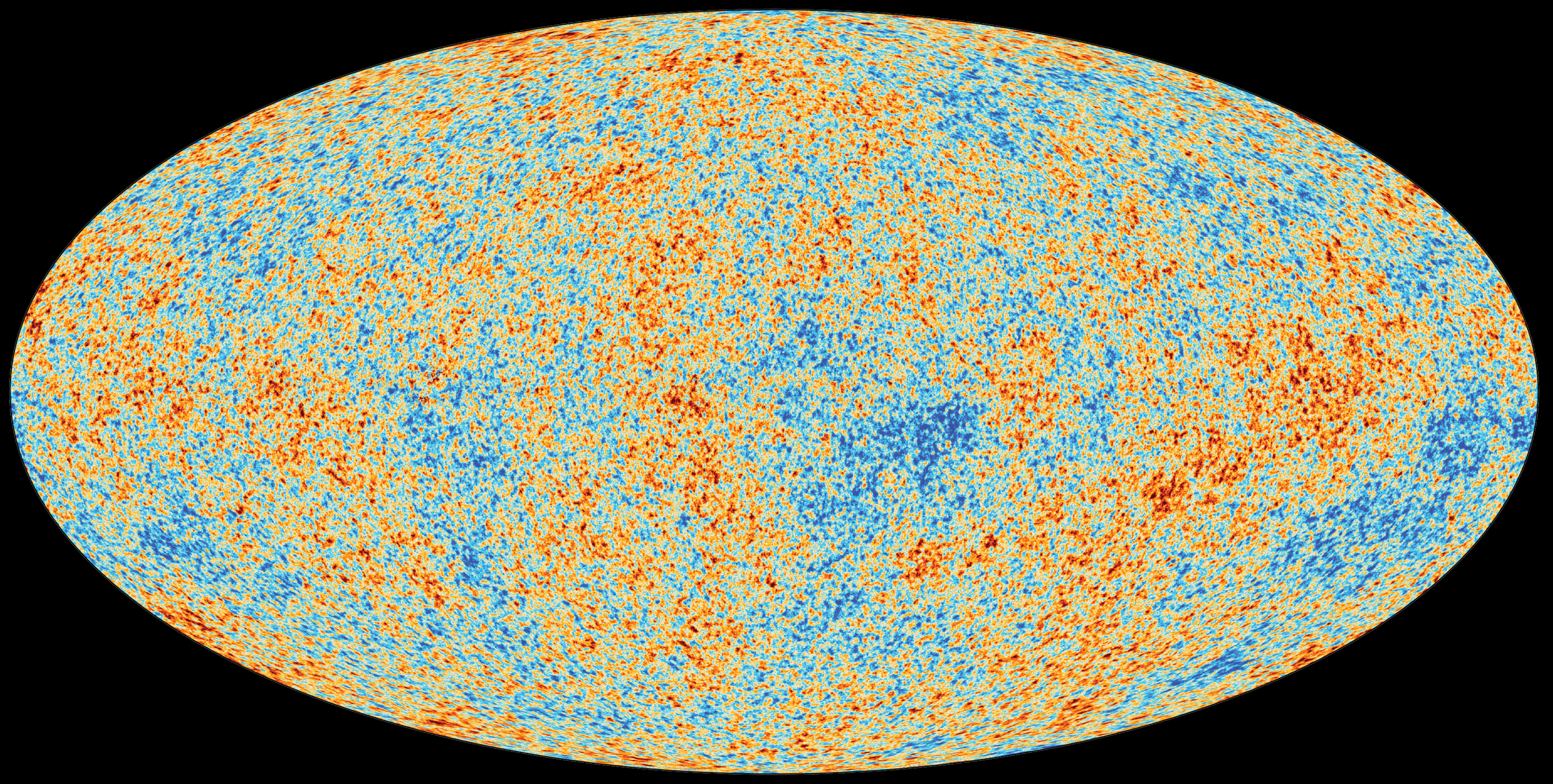
How did the structures appear from this failure to “meet up”? In the Universe there are galaxies, galaxy clusters, dwarf galaxies… The standard model, widely acknowledged and according to observations, describes a universe where small objects materialised first and, attracted by gravity, formed larger ones. The galaxies shine but the intergalactic medium does not: it contains dark matter and chemical elements such as hydrogen. Professor Matteo Viel (SISSA - Trieste) explains that “we use some atomic processes in these filaments to understand the distribution of the dark matter in the Universe and the structure formation process”. He adds: “In a way the universe is a laboratory for us and we test our theories and our models of the Universe structures against it.”
Neutrinos particles move at high velocities and hardly interact with other elements. They are remarkable since even if they are extremely common we ignore the role that they have in the play. Neutrinos, coming from astrophysical sources such as supernovae, have traveled huge distances and may be sensitive to physical phenomena handing in information on the Universe. Their mass is small compared to those of other fundamental particles. Professor Olga Mena (IFIC) calls them “dark matter hot particles” and underlines their importance in “the understanding of particle physics hierarchy, why particles have different masses”.
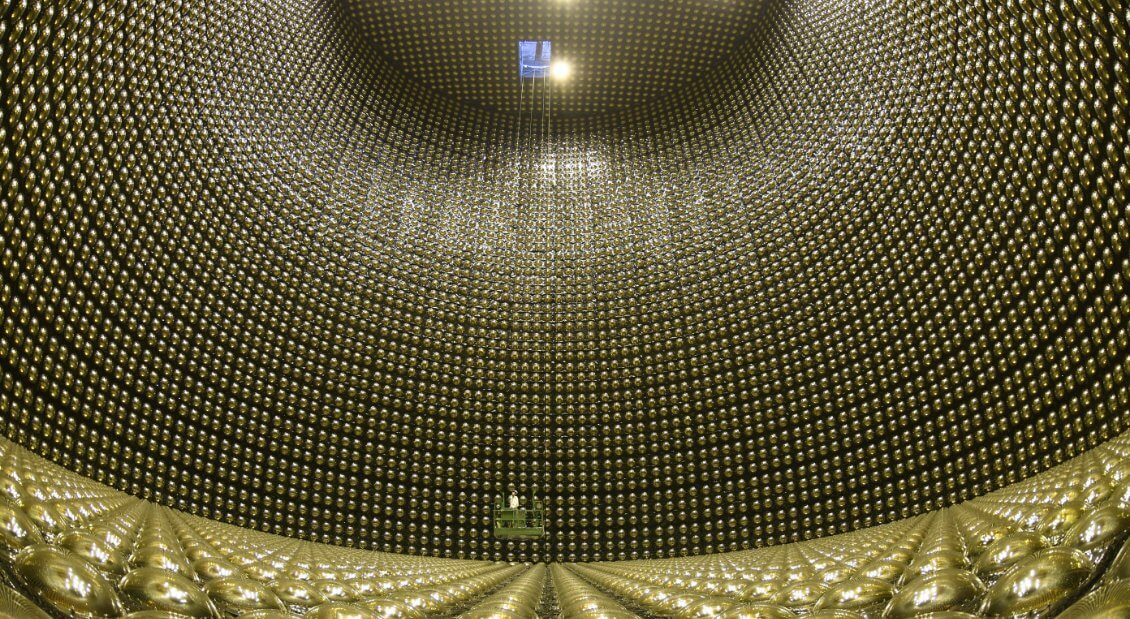
The connection between Cosmology and Particle Physics starts back in 1964 with the discovery of the Cosmic Microwave Background: the radiation generated by a 380.000 years old Universe, the first time photons could freely travel to us. From this revelation, the Big Bang becomes the accepted explanation for the Universe formation and evolution, and the standard model the one that better corresponds to the existing observations.
Dark matter
Newton’s equations describe how the Earth orbits the Sun and give the possibility to calculate their mass. The same happens with galaxies, we know their mass since it is possible to estimate the number of stars, the stellar total mass and the galaxy rotation speed. In the seventies, scientists found that the numbers did not add up, galaxy dynamics analysis revealed that a certain amount of undetected mass needed to be included in order to make sense of the observations.
It is generally accepted that dark matter constitutes 27% of the Universe, it does not produce or absorb light and it is solely detected through its gravitational influence. The denomination “dark matter” is associated to its unknown nature and the impossibility to “see” it directly. One of the proposed theories to try to explain it was related to primordial black holes. It acts like “normal” matter and models through gravity the evolution of the Universe structures. Both the theoretical models and the observations validate its reality. Supercomputers can reproduce its effects: depending on the experiment chosen parameters the structure evolves differently. It is there, and we would like to know what it is.
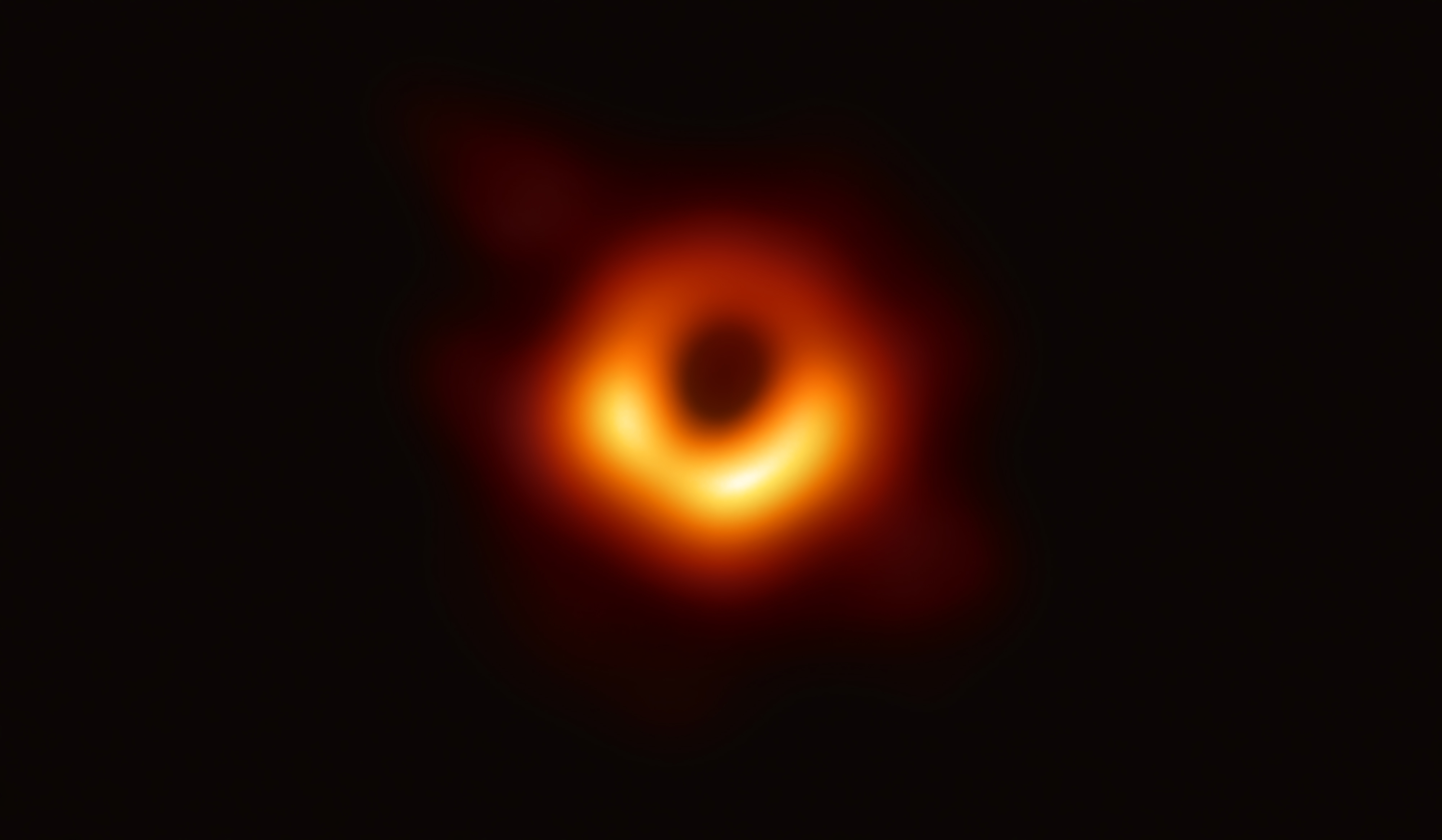
Many suggestions have been made along the years to explain this unknown: dark stars, planets, ice… We understand that it is not ordinary matter like the one that constitutes our daily world: it is not made of protons, electrons, neutrons… or by other even more fundamental particles of the physical particles standard model. Today we understand it as mass constituted by “something” still unidentified. The “bricks” of dark matter would be elemental particles still undiscovered. Physical particles and laboratory experiments have a lot to say about it.
Dark energy
Dark energy is a very different issue. In principle it would constitute 68% of the Universe and it plays a role in its expansion. In another words, the cause of the acceleration of the expansion of the Universe is called dark energy. We ignore its nature and its characteristics are highly unusual.
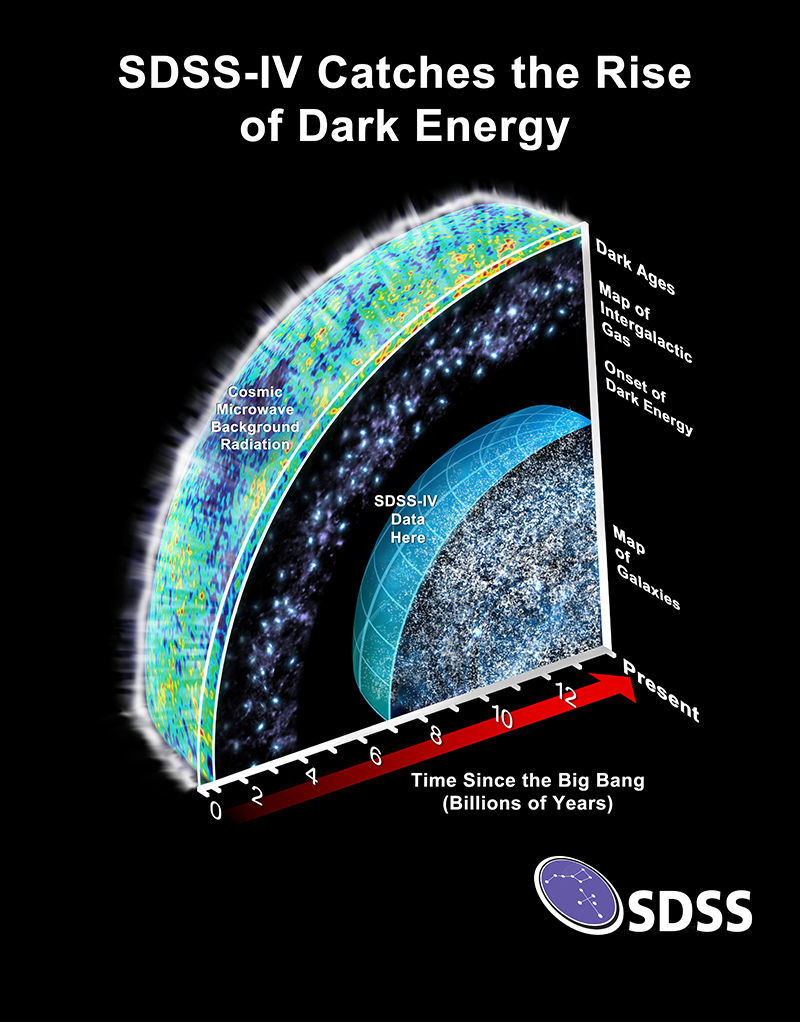
Dark energy would be equivalent to the ancient cosmological constant, proposed by Albert Einstein to explain a static universe in its gravitational equations. Later on he gave it up when Edwin Hubble demonstrated that the Universe is expanding,. Nonetheless it came back in the 90s and 2000s to explain the acceleration of the expansion. It is astronomically measured, for instance through observations showing how very distant supernovae are drifting apart.
The name may be the result of a “marketing initiative” to replace “cosmological constant”. It is a strange choice and even more confusing that the dark matter denomination. Dark energy predictions are much higher that the measured values. No explanation has been found yet which seems to match. It is even a bigger enigma than dark matter. Professor Benjamin Grinstein (University of California-San Diego) states that “when we will be able to understand why dark energy is found in such a strange amount, there will be a dynamic associated. And this dynamic is what we will be able to demonstrate. One starts wanting to understand something and when there is a theory explaining it, it predicts many other things. That is what happened with gravitational waves”.
Dark matter and dark energy do not behave similarly, there influence depends on the objects’ size. In the smaller ones, like galaxies, dark energy has hardly any influence, while dark matter does. In bigger distances, dark energy begins to be relevant and at cosmological scales both are competing
They form the dark universe, 95% of the Universe, a mystery.
An endless cognitive adventure
Understanding our Universe is both complex and fascinating. Knowing the nature of dark matter and dark energy could give us some hints on its future, whether it will go on expanding or at a certain moment it will collapse.
Centuries could pass by before being able to define what we see or the presence we detect. A good example is the Andromeda galaxy. One can distinguish it with a naked eye, without optical instruments. In the northern hemisphere there are registers of its presence one thousand years old, but its true nature remained unknown until the right technology came along and astronomers understood that through the study of some stars it was possible to determine where it was, the distance, and consequently its size.
And what about the Sun, our star. Scientists used to think that it was a ball of fire losing gravitational energy. The only problem was that, has this theory been true, our star would already have died. Actually planet Earth life expectancy was higher, it did not make any sense. At that time it was ignored that the Sun has a nuclear source of energy.
Profesor Vitor Cardoso (CENTRA/IST-Lisboa & Niels Bohr Institute-Copenhaghen) ponders: “Science is an endeavor that is useful on its own, we think about things, we find ways to rationalise the universe we see, even if we do not understand how it works now, even if we never understand it”.
What would happen to Physics if in thirty years dark matter and dark energy are still unknown? The Winter School lecturers share their thoughts.
Prof. Benjamín Grinstein, University of California-San Diego
The truth is that we do not know how or where to search. We look where technology allows us to, without knowing whether it is the right place. It could well be that dark matter does not interact with normal matter but gravitationally. That would imply that we would never see it directly, only through galaxy rotation and globular clusters as we do now.
If we do not know it in thirty years, the question is when we give up. Obviously it exists, its effects will be more evident and we will detect it with more detail in more objects.
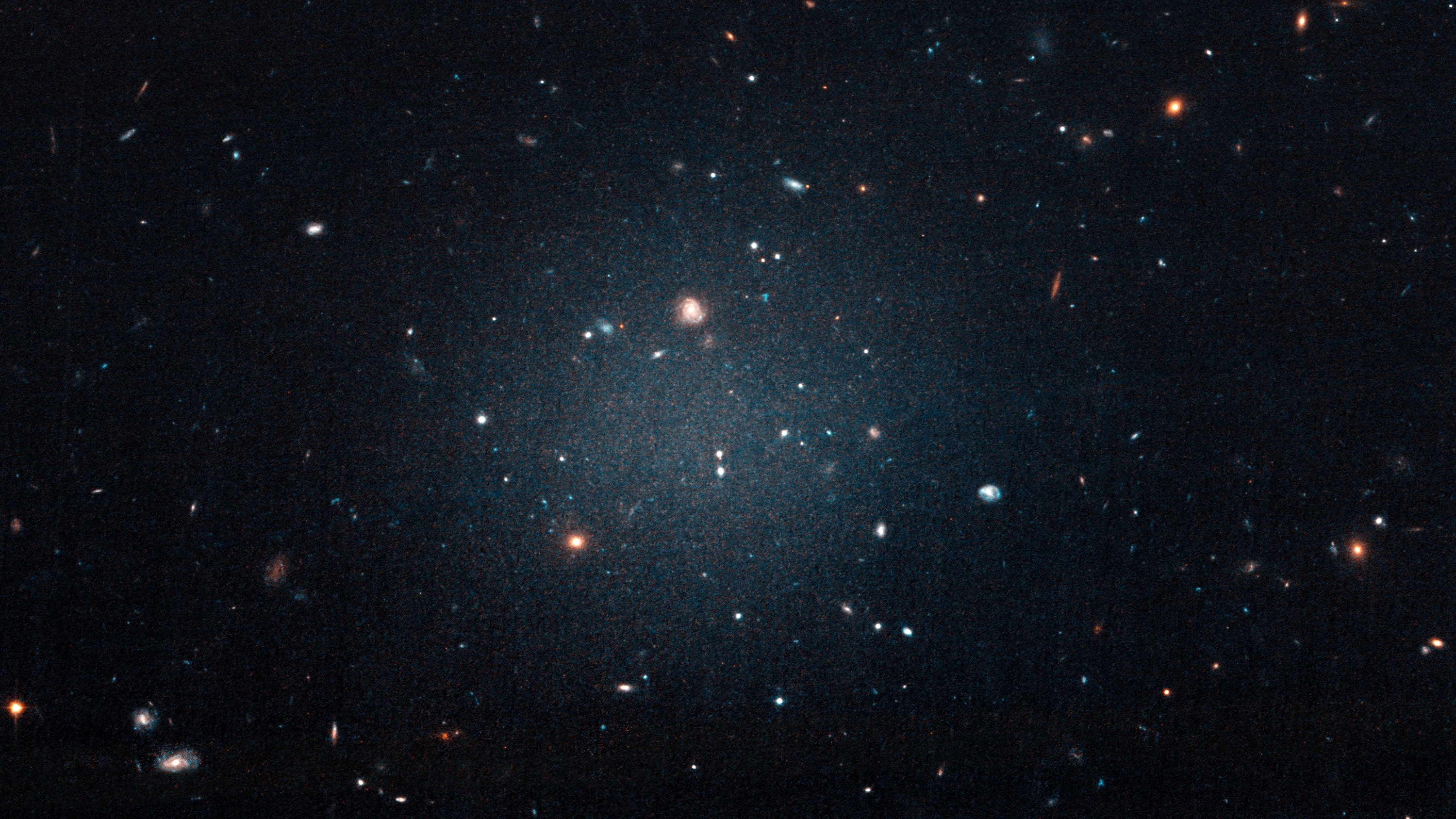
If we do not see it directly, it is not a question of lack of ideas to change the way we search, it is a matter of funding, how to convince the funders that we have to go on. How to convince young researchers of the interest of the question and have them believe that they can find the answer after forty years looking unsuccessfully.
We are completely lost with dark energy. I have not heard any good idea to understand its nature, it is only studied through its effects. Would somebody have an idea to directly test its nature? In my opinion progress would come from modelisation.
Prof. Kfir Blum, Weizmann Institute of Science – Rehovot
I think that there is an excellent chance that we will not detect dark matter in thirty years. There is an excellent chance that we will not detect it in a thousand years. It is possible that we do not have now the technology either mathematical or experimental to understand what dark matter is.
What will happen to Physics? Dark matter is a very difficult problem, but we could be lucky and in a few decades solve it. There is no reason to think right now that this will be the case. Dark energy, like dark matter, is an spectacular question in Physics. We are lucky to have such spectacular questions to be fighting against. Since the fight may be long, we must enjoy the road by solving easier questions we encounter on the way.
For instance, globular clusters are arranged in a certain pattern in some galaxies. I think there is a chance we could really understand this pattern. If we do, there is certain reason to think we will learn about dark matter. Even if we do not learn about dark matter, we will be making progress. We want to understand objects, systems, we do not want to write theories on dark matter that are completely hopeless.
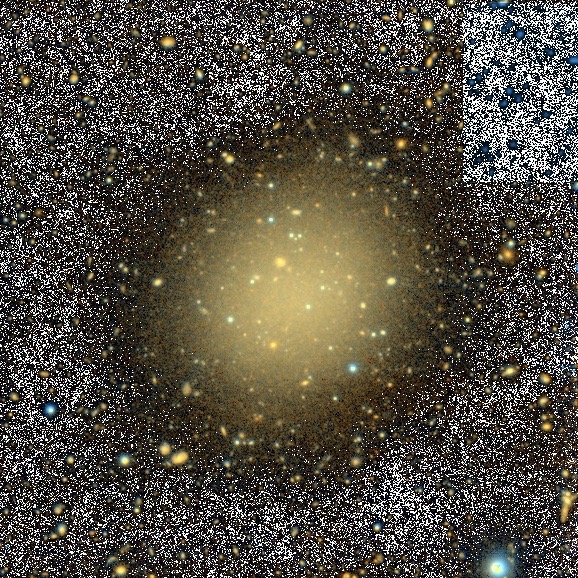
I am not worried at all about what will happen in thirty years in Physics, I hope we will not just invent futile new theories. Our job is to solve questions in Physics. As long as we do that, and we should be aware of the dark matter question, eventually we will solve it in the next thirty year or we will take a thousand years. Each of us should solve questions. Nothing will happen to Physics.
Prof. Olga Mena, IFIC
Even if we have not elucidated the fundamental nature of dark matter and dark energy, we will certainly know much more than today. They are very important questions that should not be put aside until having the perfect explanation.
Cancer will not be eradicated in thirty years but research will go on and on the way do fantastic discoveries with other benefits. The same in the dark matter and dark energy fields. I hope that some signal will happen but even if it is not the case there will be progress. Besides new and very big technological structures will be built such as telescopes and super accelerators. They will give lots of information, the question will also be how to deal with such an amount of data, bigger than today’s Internet traffic.
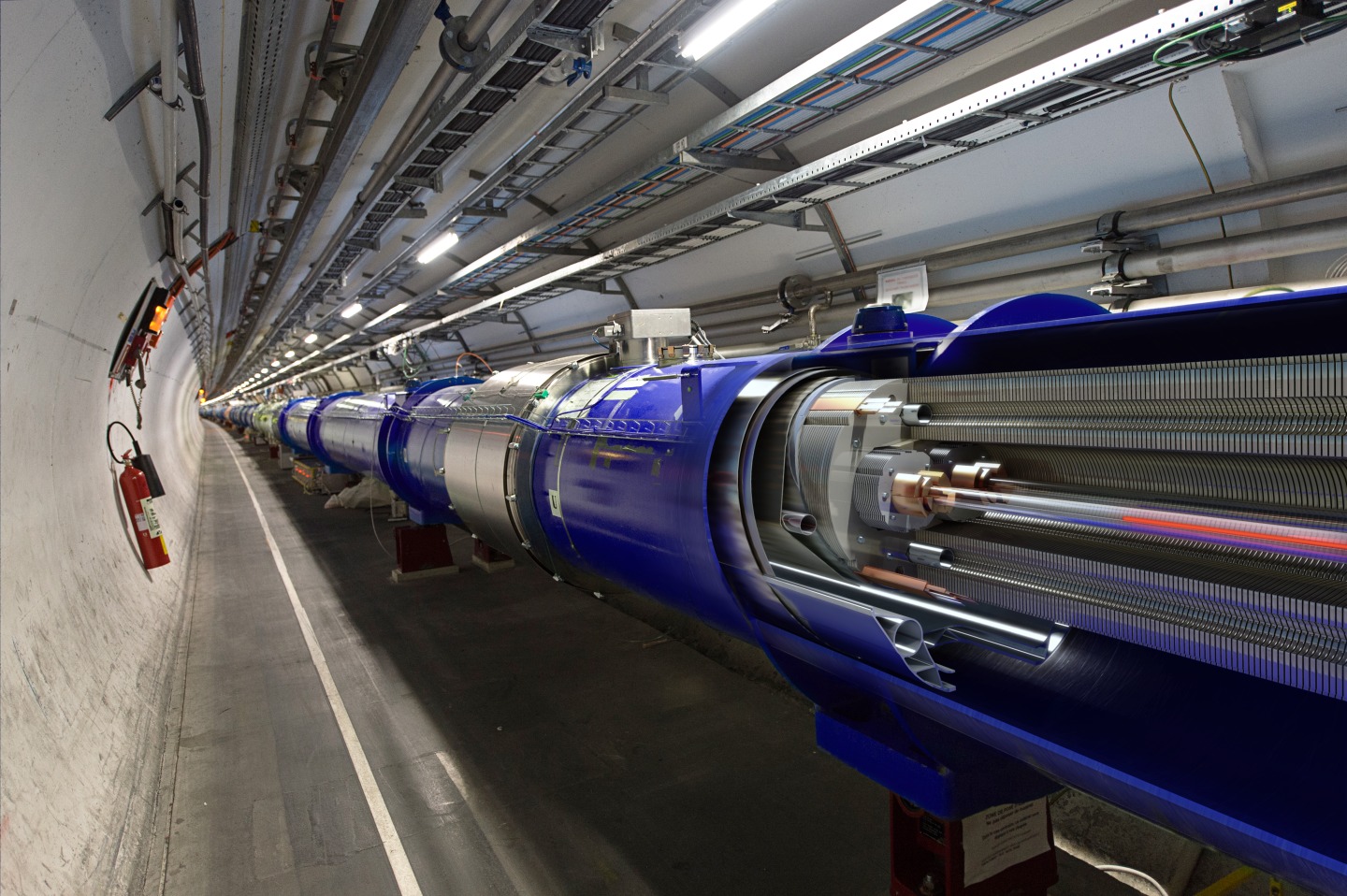
In my opinion Physics has to be there and will be there. Understand the Universe origin and destiny are fundamental questions to face and solve, or at least delimit or try to solve. Physics future is not in danger.
Prof. Vitor Cardoso, CENTRA/IST - Lisboa & Niels Bohr Institute – Copenhaghen
It is embarrassing because it is a problem that has been with us 50-70 years and we have not made any progress at all. It may be because we are not good enough, some new Einstein needs to come along and give us a totally unexpected solution. I used to have the view point that we are just doing science in the wrong way nowadays, we are tackling small problems, always concerned about our career and our publications. My position was “let’s have a more noble attitude towards science”. And the answer will come along from a guy who is not concerned about publishing a number of papers in a year.
It could also be that the Universe is not making our life easy, that we are doing what we should, trying to go through all the options and trying to rule them out, for instance: is dark matter a weekly interacting particle? If in thirty years we do not solve the dark matter problem, I do not think it is going to change anything.
Public perception will depend on how we communicate, they can understand that it is hard. There is also the fact that millions of euros have been invested in technology, we are building detectors today to be operated in forty years by the next generation. Society may think that it is too much money to spend in something we do not know if it is going to work. I believe that a society that chooses that is a very poor society but it is a real danger.
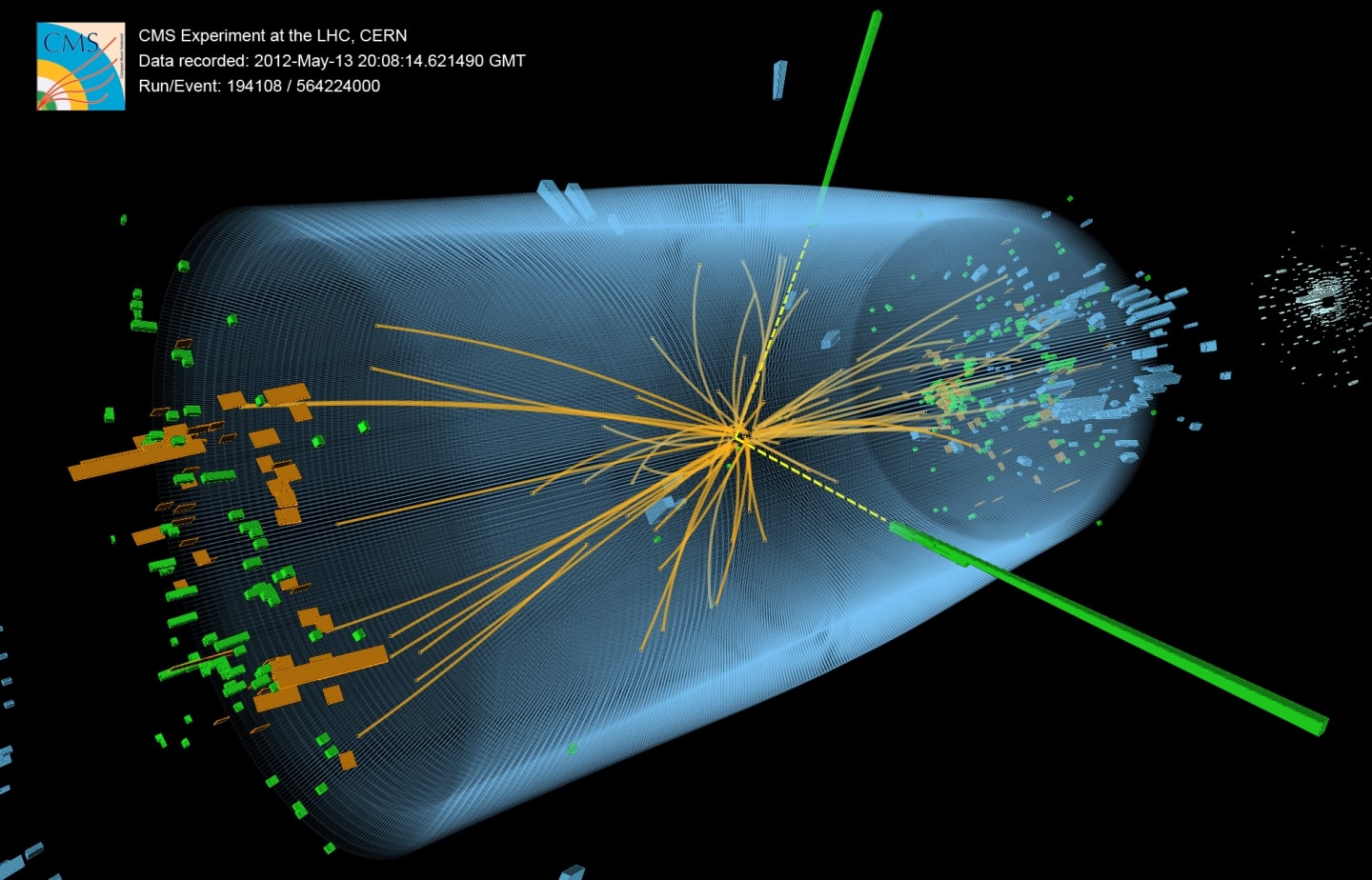
Prof. Matteo Viel, SISSA – Trieste
There could be candidates for dark matter like scalar fields which are going to be incredibly difficult to detect, both in laboratories or in the Universe, for instance there could be regions of the parameter space of theories that are very difficult to test, also in thirty years from now. However, if this is going to be the case ( no dark matter detection within thirty years from now), I believe we should consider more seriously modification of gravity at the scales of cosmic structures.
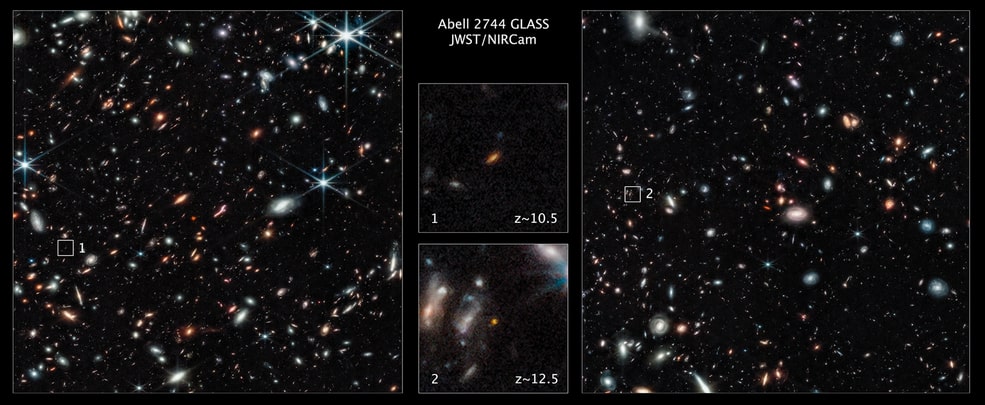
I believe that there is a wide set of key experiments in the next five years that are likely to tell us whether or not the dark energy is dynamical or a cosmological constant. If an evidence for a dynamical behaviour is found this is going to be an extraordinary discovery that will trigger further theoretical studies to interpret the results. However, if in thirty years from now we will not discover dynamical dark energy and not find any connection between cosmological constant and more fundamental theories what it is likely is that we will "accept" this as an initial condition or realization of Einstein equation that the Universe is fulfilling.
My gratitude to the Winter School teachers for sharing not only their knowledge about the Universe, but also their ignorance.
More information
Winter School press release and images
Winter School website
https://research.iac.es/winterschool/2022/
VOCES - Malcolm Longair
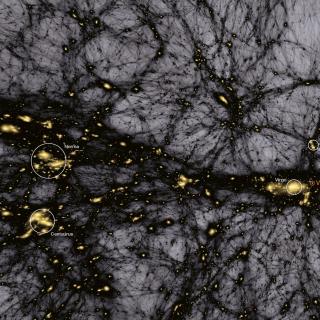
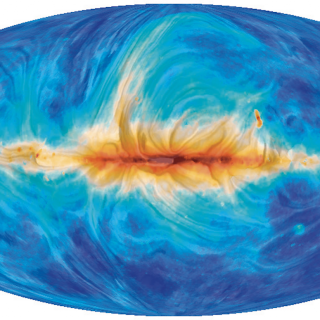
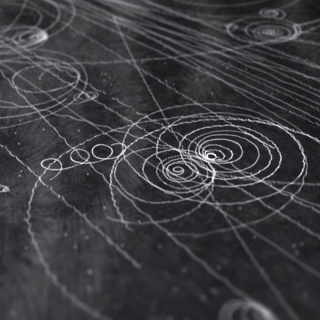
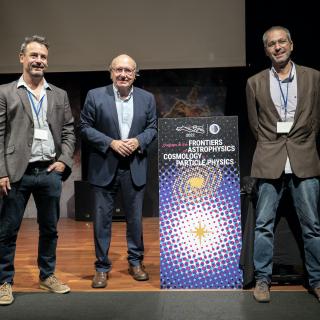